Fundamentals to understand reality from the perspective of humanity
Is energy a physical entity or an abstract notion to quantify the basic reality like material fundamental Forces?
Energy is a physical quantity that is used to quantify the ability to do work. It is a scalar quantity, meaning it has only magnitude and no direction. The Energy can exist in various forms, such as kinetic energy, potential energy, thermal energy, and so on. It is also considered as a conserved quantity, meaning the total energy in a closed system remains constant. It is a fundamental concept in physics and is used to describe the behavior of matter and energy in various systems.
.
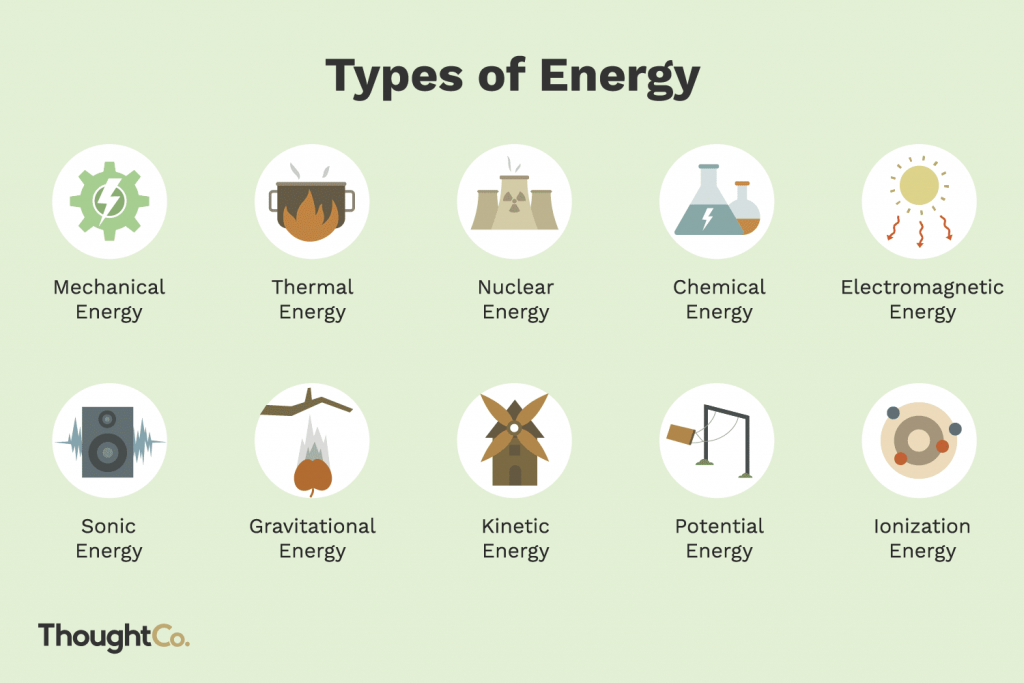
What are other forms of energy? How the chemical energy is converted to physical energy by mitochondria in animals?
Other forms of energy include:
1- Mechanical energy, which is the sum of kinetic and potential energy of an object.
2- Thermal energy, which is the internal energy of a substance due to its temperature.
3- Nuclear energy, which is the energy stored in the nuclei of atoms.
4-Chemical energy, the energy that absorbed or released in a chemical reaction.
5-Electromagnetic, energy, which is the energy associated with the movement of electric charges, the energy du to flow of electron and Radiant energy, which is the energy associated with electromagnetic waves, such as light.
6-Sonic Energy, the energy of sound waves, the energy transmitted by sound wave.
7-Gravitational energy, the energy du to gravitational pull among objects.
8- ionization energy, the energy that is the form charge particles, actual a form electromagnetic energy.
9-Kinetic energy, the energy du to the motion of an objects.
10 Potential energy, that is held due the position of an object relative to the other objects.
In animals, chemical energy is stored in the bonds of molecules such as glucose. The process by which this chemical energy is converted into a form that can be used by cells is called cellular respiration. This process takes place in the mitochondria, which are organelles found in the cells of animals and other eukaryotic organisms. During cellular respiration, glucose and oxygen are consumed, and carbon dioxide and water are produced as waste products. The energy released during this process is used to produce a molecule called adenosine triphosphate (ATP), which is the primary source of energy for cells.
** Molecule
:Energy released by the oxidation of glucose is in the form of heat or what?::
The energy released by the oxidation of glucose during cellular respiration is in the form of chemical energy. Specifically, it is in the form of the energy stored in the chemical bonds of adenosine triphosphate (ATP), which is the primary source of energy for cells. The process of oxidation of glucose in the mitochondria generates ATP by transferring electrons from glucose to oxygen through a series of redox reactions, which also generate heat as a byproduct.
The overall process of cellular respiration can be divided into two main stages:
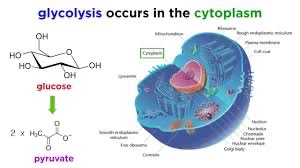
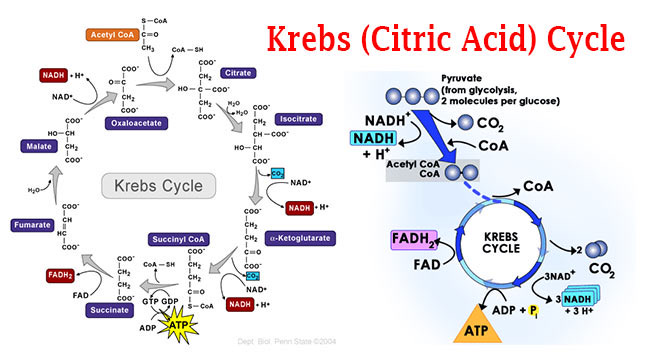
- Glycolysis takes place in the cytoplasm, where a single glucose molecule is broken down into two molecules of pyruvate and generates two ATP molecules.
- The citric acid cycle takes place in the mitochondria and completes the oxidation of glucose. It generates high-energy electrons that are passed through the electron transport chain, creating a proton gradient that drives the production of ATP through ATP synthase. The citric acid cycle also generates CO2 and water as byproducts.
Overall, the oxidation of glucose in the mitochondria generates a large amount of ATP and some heat as a byproduct.
Energy cycles in detail::
- 1-Cellular respiration is the process by which cells convert glucose and oxygen into carbon dioxide, water, and energy (ATP). This process occurs in the mitochondria of eukaryotic cells and can be divided into three stages: glycolysis, the citric acid cycle, and the electron transport chain. 2- Photosynthesis is the process by which plants, algae, and some bacteria convert light energy into chemical energy in the form of glucose. This process occurs in the chloroplasts of plant cells and can be divided into two stages: the light-dependent reactions and the light-independent reactions (Calvin cycle). 3- The Nitrogen cycle is the process by which nitrogen is converted into various forms that can be used by living organisms. Nitrogen gas (N2) is converted into ammonia (NH3) by nitrogen-fixing bacteria, which is then converted into nitrite (NO2) and nitrate (NO3) by other bacteria. These forms of nitrogen can be taken up by plants and used to make proteins and other important molecules. 4- The Water cycle is the process by which water evaporates from the surface of the earth, forms clouds, and falls back to the surface as precipitation. This water can then be taken up by plants, evaporate again, or flow into rivers and oceans. 5- The Carbon cycle is the process by which carbon is exchanged between the atmosphere, oceans, and living organisms. Carbon dioxide (CO2) is taken up by plants during photosynthesis, and can also be dissolved in the oceans. It can also be released back to the atmosphere through respiration and the burning of fossil fuels. It’s worth noting that these cycles are interconnected and rely on each other. Understanding these cycles is important for understanding how energy and matter move through the biosphere, and how changes to one cycle can impact the other.
High energy electrons when passed through electron chain, how that is converted into a physical activity like movements or pulling and pushing?
When high-energy electrons are passed through the electron transport chain, they lose some of their energy to a series of protein complexes. These protein complexes use the energy from the electrons to pump protons (hydrogen ions) across the inner membrane of the mitochondria. This creates a gradient of protons across the membrane, with a higher concentration of protons on one side of the membrane than the other. This proton gradient is a form of potential energy.
The energy stored in this proton gradient is then used to power the production of ATP. Specifically, the energy is used to drive the movement of protons back across the membrane through a protein called ATP synthase. As the protons move back across the membrane, they drive the rotation of a part of the ATP synthase called the rotor. This rotation generates mechanical energy which is used to power the phosphorylation of ADP to ATP.
The ATP produced by this process can then be used by cells to power various cellular processes such as muscle contraction, which leads to movement, or the transport of molecules across membranes, which is necessary for many physiological processes.
In summary, the energy stored in the proton gradient is converted into mechanical energy which is used to generate ATP. This ATP can then be used to power various physical activities such as movements, pulling and pushing and other cellular activities.
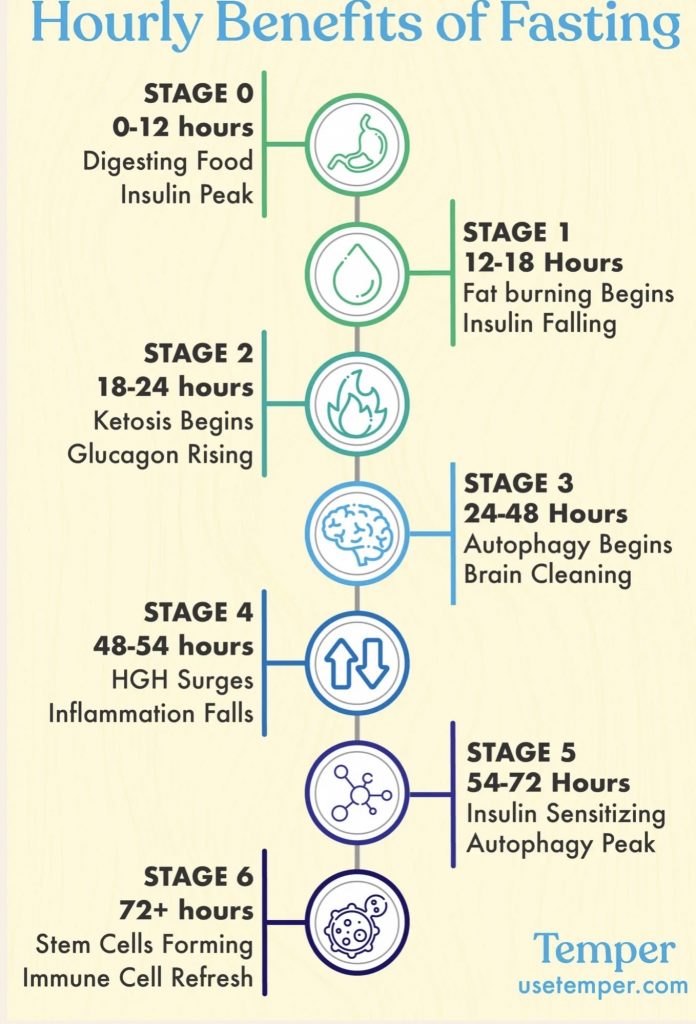

What happens if the glucose supply is blocked by fasting for longer period of time?
If the glucose supply is blocked by fasting for a longer period of time, the body will begin to use alternative sources of energy. The body can obtain energy from fat stores by breaking down fatty acids and ketone bodies, which can be used as an energy source for the brain. This process is called ketosis.
During the first 24-48 hours of fasting, the body will continue to use glucose as the primary energy source, but will begin to break down glycogen stores in the liver to provide glucose for the brain and other tissues. After the glycogen stores are depleted, the body will begin to break down fatty acids to produce ketone bodies, which can be used as an energy source by the brain and other tissues.
The brain, which normally uses glucose as its primary energy source, can also use ketone bodies as an energy source. This is why the brain can function normally during periods of fasting or low-carbohydrate diets.
It’s worth noting that, depending on the duration of the fasting, this process may have some negative consequences on the body. For example, if the fasting is too long, it can lead to muscle wasting, nutrient deficiencies, and other health problems. Therefore, it is important to consult a healthcare professional before attempting a fasting regimen.
Fasting also triggers the conserved program like autophagy
Yes, fasting can also trigger a conserved program called autophagy. Autophagy is a process by which cells break down and recycle their own components, including damaged or unnecessary proteins and organelles. Autophagy plays an important role in maintaining cellular homeostasis and can help protect cells against stress and damage.
During periods of fasting, autophagy is activated as a way for cells to conserve energy and provide building blocks for the synthesis of new molecules. The decrease in the availability of nutrients, especially glucose, leads to an increase in the level of a protein called AMPK, which acts as a signaling molecule to stimulate autophagy.
Autophagy helps cells to maintain energy homeostasis by breaking down and recycling damaged or unnecessary proteins and organelles, which can be converted into energy and nutrients. Autophagy also plays a role in the removal of misfolded proteins and damaged organelles, which can prevent the accumulation of harmful material that can lead to cell damage and disease.
It’s also worth noting that autophagy can be triggered by other stimuli such as exercise, caloric restriction and some drugs. Studies have shown that autophagy may have beneficial effects on health, including increasing lifespan and reducing the risk of certain diseases. However, excessive autophagy may also be harmful, so it’s important to maintain a balance in the process.
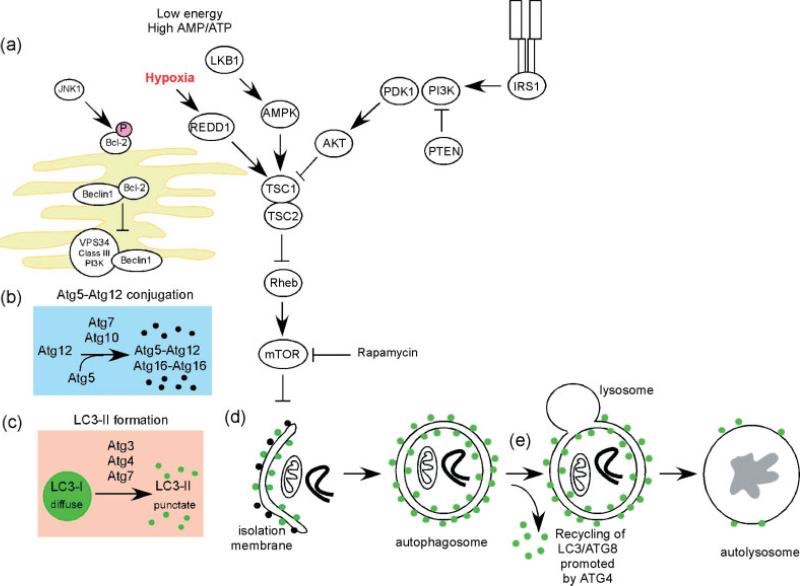

For detail click Autophogy
What are the other conserved programs to helps us to have a batter survival chance and how those can be triggered? There are several other conserved programs that help organisms to have a better survival chance. Some of these programs include:
- Stress response: The stress response is a conserved program that allows organisms to adapt to various forms of stress, such as heat, cold, or injury. This response is mediated by hormones such as cortisol and adrenaline, which help to increase energy production and reduce inflammation. Stress response can be triggered by various stimuli such as changes in temperature, injury, or infection. 1- DNA repair: DNA damage is a constant threat to cells, and organisms have evolved a number of mechanisms to repair DNA damage. DNA repair can be triggered by various stimuli such as exposure to UV light, ionizing radiation, or chemical agents. 2- Apoptosis: Apoptosis is a process of programmed cell death that plays a crucial role in the development and maintenance of multicellular organisms. Apoptosis can be triggered by various stimuli such as DNA damage, viral infections, or exposure to certain toxins. 3- Immune response: The immune response is a complex system that helps to protect organisms from infection and injury. The immune system can be triggered by various stimuli such as the presence of foreign substances, injury, or infection. 4- Senescence: Senescence is a conserved program that leads to the decline of the organism’s ability to reproduce and respond to stress. It can be triggered by various stimuli such as DNA damage, telomere shortening, and oxidative stress. It’s worth noting that these programs are not mutually exclusive and can interact with each other to provide a survival advantage. Additionally, these programs can be triggered by different stimuli, and the response can vary depending on the organism and the specific conditions.
Overall, these conserved programs are a crucial part of the survival of an organism, and they help to ensure that the organism can adapt to changing environments and survive in the face of various challenges.
How these conserved survival programs evolved and what were the driving forces to select conserved programs?
Give all the detail of contributing factors in evolution The conserved survival programs that you’ve mentioned have evolved over time through the process of natural selection. Natural selection is the process by which certain traits become more or less common in a population over generations based on their effects on the survival and reproduction of the individuals that possess them.
The driving force behind the evolution of these conserved programs is the need for organisms to survive and reproduce in their environment. In order to do so, organisms must be able to adapt to changing conditions and respond to various challenges such as injury, infection, or environmental stress. Traits that help organisms to survive and reproduce in their environment are more likely to be passed on to future generations, while traits that do not are less likely to be passed on. Over time, this process leads to the evolution of traits that are beneficial for survival and reproduction.
Several factors contributed to the evolution of these conserved programs:
1- Environmental pressures: the environment in which organisms live plays a crucial role in shaping their evolution. Environmental pressures such as temperature changes, predation, and competition for resources can drive the evolution of traits that help organisms to survive and reproduce in that environment.
2- Genetic variation: genetic variation is the raw material of evolution. Mutations in DNA can lead to the creation of new genetic variants, and natural selection can act on these variants to produce new traits.
3- Mutation and genetic drift: Mutations are the source of genetic variation and genetic drift is the random fluctuation of allele frequencies in a population. Both of these processes can contribute to the evolution of conserved programs.
4- Gene regulation and epigenetics: Gene regulation and epigenetics play an important role in the evolution of conserved programs. The regulation of gene expression can affect how a trait is expressed, and epigenetics can affect how a trait is inherited.
5- Developmental and evolutionary constraints: developmental and evolutionary constraints affect the evolution of conserved programs by limiting the range of possible solutions to a particular problem.
In summary, conserved survival programs have evolved over time through the process of natural selection as a response to environmental pressures, genetic variation, and other factors. These programs have enabled organisms to adapt to their environment and survive in the face of various challenges.
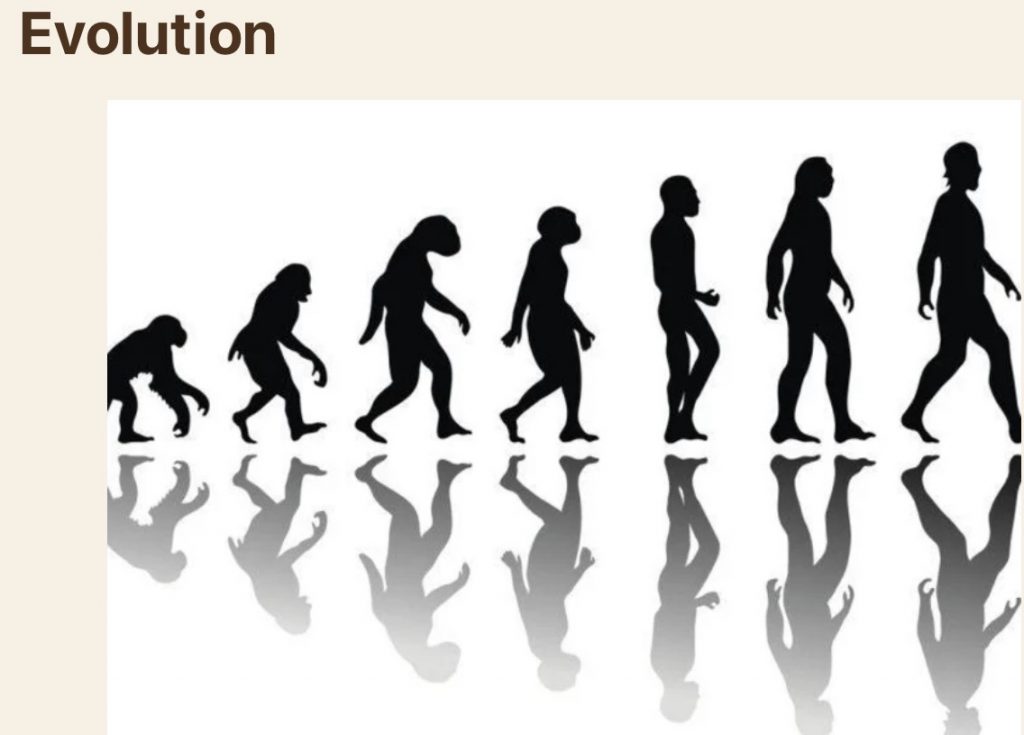
:Evolution::
Vertical and horizontal evolution in detail with example
Vertical evolution, also known as Darwinian evolution, refers to the gradual accumulation of genetic changes over time through the process of natural selection. This process occurs over many generations and results in the gradual accumulation of genetic variations that are passed on from parent to offspring. The genetic variations that are passed on are those that increase the fitness of the organism, meaning that they increase the organism’s chances of survival and reproduction. This gradual accumulation of genetic variations leads to the evolution of new species over time.
An example of vertical evolution is the evolution of the human eye. The eye is a complex organ that allows us to see, and it is thought to have evolved over millions of years through a series of small genetic changes. The earliest eyes were probably simple light-sensitive cells that allowed organisms to detect changes in light levels. Over time, these simple eyes gradually evolved into more complex eyes, with the development of lens, retina, and other structures that allow us to see in greater detail.
Horizontal evolution, also known as lateral gene transfer, refers to the movement of genetic material between organisms that are not parent and offspring. This can occur through a variety of mechanisms, such as horizontal gene transfer, hybridization, and transduction. Horizontal evolution can lead to the rapid spread of beneficial traits through a population, and can also lead to the formation of new species.
An example of horizontal evolution is the evolution of antibiotic resistance in bacteria. Bacteria can acquire resistance to antibiotics through horizontal gene transfer, where they can pick up a piece of DNA that encodes for resistance from another bacteria. This can happen through various mechanisms such
Such as such as transformation, transduction, and conjugation. For example, a bacteria that is naturally resistant to an antibiotic may transfer that resistance gene to a different bacteria, which then becomes resistant as well. This can happen quickly, and can lead to the rapid spread of antibiotic resistance through a population of bacteria. This process can be a significant challenge in medicine, as the widespread use of antibiotics has led to the evolution of antibiotic-resistant bacteria that are difficult to treat.
Another example of horizontal evolution is the evolution of eukaryotic cells through the endosymbiotic theory. According to this theory, eukaryotic cells, which are cells with a nucleus and other membrane-bound organelles, evolved from the fusion of different prokaryotic cells. This event allowed for the formation of new organelles such as mitochondria and chloroplasts which are crucial for the survival of eukaryotic cells. This theory suggests that mitochondria and chloroplasts were once free-living prokaryotic cells that formed symbiotic relationships with other cells, eventually becoming a part of the host cell.
In summary, vertical evolution refers to the gradual accumulation of genetic changes over time through the process of natural selection, while horizontal evolution refers to the movement of genetic material between organisms that are not parent and offspring. Both processes play an important role in the evolution of organisms and can lead to the formation of new species and new traits.
The role of epigenetic in evolution
Epigenetics is the study of heritable changes in gene function that do not involve changes to the underlying DNA sequence. Epigenetic mechanisms play a role in the regulation of gene expression and can affect the development and evolution of organisms.
Epigenetic modifications, such as methylation and acetylation, can affect the accessibility of the DNA to the transcription machinery and therefore, can silence or activate certain genes. These modifications can be passed on through cell division and even through generations.
Epigenetics plays a crucial role in the development of organisms by determining which genes are turned on and off in different cells and at different stages of development. For example, during embryonic development, certain genes are turned on and off in a precise sequence to create the different cell types that make up the body. Epigenetics also plays a role in aging and disease, as well as in the response to environmental factors.
In the context of evolution, epigenetics can also play a role. The ability of epigenetics to respond quickly to environmental changes and pass on the beneficial changes to future generations can provide a mechanism for rapid adaptation to new environments. Epigenetic modifications can also affect the rate of evolution by altering the way in which natural selection acts on the genome.
For example, some research suggests that epigenetic modifications may have played a role in the evolution of different human populations by allowing them to adapt to different environmental conditions, such as changes in diet or altitude. Additionally, epigenetic modifications may have played a role in the evolution of complex traits, such as behavior and cognition.
It’s worth noting that the role of epigenetics in evolution is still a topic of active research, and the exact mechanisms and extent of its contribution is still unclear. However, it is becoming clear that epigenetics plays a role in the regulation of gene expression and can affect the evolution of organisms in various ways.
What is a gene drive and how it helps us to solve biological problem like to eliminate malaria?
A gene drive is a genetic mechanism that can spread a particular gene or set of genes through a population more quickly than would be expected under normal inheritance patterns. Gene drives can be used to spread beneficial traits, such as resistance to disease, or to eliminate harmful traits, such as those that allow mosquitoes to transmit malaria.
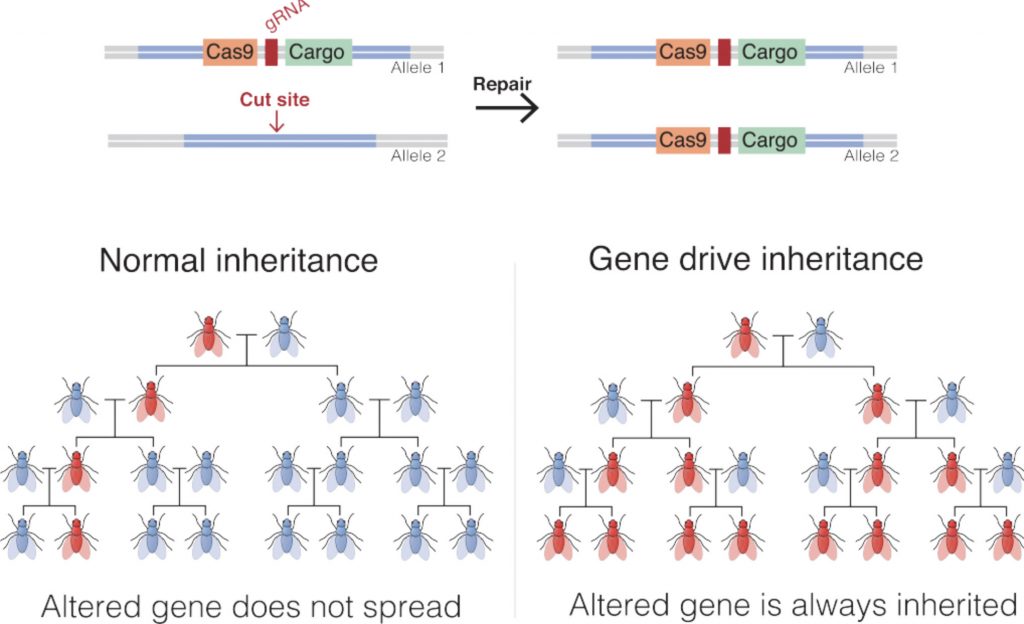

One way gene drives work is by using a genetic construct called a homing endonuclease, which is a type of enzyme that can cut DNA at a specific location. When the homing endonuclease cuts the DNA, it creates a double-stranded break. When the cell repairs the break, it can use the genetic construct as a template, which results in the insertion of the desired gene into the genome.
The use of gene drives to eliminate malaria is still in research and development stage, but it has been proposed as a promising approach to controlling the spread of the disease. By introducing genes that make mosquitoes resistant to the malaria parasite into wild mosquito populations, researchers hope to reduce the number of mosquitoes that can transmit the disease. This would ultimately lead to a reduction in the number of malaria cases, and could potentially help to eliminate the disease altogether.
It’s worth noting that there are still many scientific and ethical challenges to be addressed before gene drives can be used in real-world scenarios, and more research is needed to understand the full implications of this technology. Additionally, researchers are also looking at other methods to control the spread of malaria such as using genetically modified mosquitoes that can’t transmit the disease, or releasing lab-raised mosquitoes that are resistant to the disease.
Other implications of gene drive with examples
The use of gene drives has the potential to have a wide range of implications, both positive and negative. Some of the potential implications include:
- Environmental impact: Gene drives have the potential to drastically change the genetic makeup of wild populations, which could have unintended consequences for ecosystems and other organisms that rely on those populations. For example, the elimination of a certain species could disrupt the food chain and cause cascading effects on other species.
- Economic impact: Gene drives have the potential to impact agriculture and food production. For example, the elimination of crop pests could increase crop yields, but it could also disrupt existing pest control methods and lead to new pest outbreaks. 1- Ethical implications: Gene drives raise a number of ethical concerns, such as the possibility of unintended consequences, the possibility of creating “genetic pollution,” and the impact on the rights of people and other organisms. 2- Social impact: Gene drives have the potential to impact human health, particularly in the context of disease control. For example, if gene drives are used to eliminate disease vectors such as mosquitoes, they could have a significant impact on public health in developing countries where these diseases are prevalent. 3- Socio-economic impact: Gene drives have the potential to have socio-economic impact by affecting the livelihoods of people who depend on the affected organisms for their survival. For example, if gene drives are used to eliminate a certain crop pest, it could affect the livelihoods of farmers who rely on that crop for their income. It’s worth noting that these are just a few examples of the potential implications of gene drives, and the full range of implications is not yet known. Additionally, the use of gene drive is a complex topic that raises many ethical and societal questions, and it is important to consider the potential risks and benefits before implementing any gene drive-based approach.
Other promising biotechnologies which are going to be blessings for humanity if used ethically and cautiously
There are several other biotechnologies that have the potential to be blessings for humanity if used ethically and cautiously. Some of these include:
1- CRISPR-Cas9:
CRISPR-Cas9 is a powerful gene editing tool that allows scientists to make precise changes to the genome.
This technology has the potential to be used to treat genetic diseases, create more resilient crops, and even eliminate certain diseases. 2- Synthetic biology: Synthetic biology is an emerging field that combines engineering principles with the study of biological systems. This technology has the potential to be used to produce new medicines, biofuels, and even create new organisms with specific functions. 3- Stem cell therapy: Stem cell therapy is a promising field that involves using stem cells to repair or replace damaged or diseased cells. This technology has the potential to be used to treat a wide range of diseases, including cancer, heart disease, and spinal cord injury. 4- Bioprinting: Bioprinting is a technology that uses 3D printing techniques to create functional biological tissue. Bioprinting has the potential to be used to create replacement organs and other tissue for transplants, and to study the development of diseases. 5- Genome-wide association studies (GWAS): GWAS are a powerful tool that allows scientists to identify genetic variations that are associated with certain diseases. This technology has the potential to be used to understand the genetic basis of many diseases and to identify new drug targets. It’s worth noting that these technologies are all still in the early stages of development and have not yet been fully developed and implemented in the real world. Additionally, these technologies raise many ethical and societal
What do you think that by using these biotechnologies, can we achieve extended health spans or longevity and in what time frame and at what extents?
The use of biotechnologies such as CRISPR-Cas9, synthetic biology, stem cell therapy, bioprinting, and genome-wide association studies has the potential to improve human health and extend health spans. However, it is important to note that these technologies are still in the early stages of development and it is difficult to predict exactly when and to what extent they will be able to achieve extended health spans and longevity.
CRISPR-Cas9 and other genome editing technologies have the potential to be used to treat genetic diseases and to reduce the risk of age-related diseases. However, it will take time to fully understand the risks and benefits of these technologies and to develop safe and effective therapies.
Synthetic biology has the potential to be used to create new medicines and biofuels, which could improve human health and extend health spans. However, it will take time to fully understand the risks and benefits of these technologies and to develop safe and effective therapies.
Stem cell therapy has the potential to be used to repair or replace damaged or diseased cells, which could improve human health and extend health spans. However, it will take time to fully understand the risks and benefits of these technologies and to develop safe and effective therapies.
Bioprinting has the potential to be used to create replacement organs and other tissue for transplant..